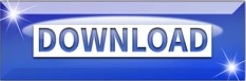
The flexibility inherent in that design allowed the addition of multiband “pushframe” imaging and time-delayed integration (TDI), described in Sect. To optimize the camera design within constrained resources, the camera electronics design is based on that developed earlier for the Mars Science Laboratory (MSL) mission (Edgett et al. A single methane filter is not a comprehensive cloud detector, but it is the optimal choice given severe constraints of cost, mass, camera sensitivity, and compatibility with the basic camera design. High clouds and hazes stand out in 889 nm images, since they reflect more sunlight than the absorbing gas around them. This is addressed by having a filter at 889 nm, which is an absorption band of methane, a well-mixed gas in Jupiter’s atmosphere. Since clouds are both signal and noise for these instruments, the ability to image clouds becomes an additional requirement for Junocam. These include the microwave radiometer (MWR), the ultraviolet imaging spectrograph (UVS), and the Jovian infrared auroral mapper (JIRAM). The third opportunity is to serve as the “eyes” in visible and near-infrared light for other remote sensing instruments on Juno. It leads to a resolution requirement of 3 km/pixel at perijove, when the spacecraft is near the equator, and 50 km/pixel when the spacecraft is over the pole. Another opportunity is studying the equatorial region at ten times higher spatial resolution than that of Voyager, Galileo, and Cassini. This in turn leads to a field of view requirement of about 60 degrees. Observing the pole is one such opportunity, both for EPO and science, and it leads to the requirement of imaging the entire polar region in three colors (red, green and blue) as the spacecraft passes over the poles ☑ h from closest approach. The science and EPO objectives evolved from trade studies balancing the unique imaging opportunities arising from the Juno orbit with the constraints of cost and spacecraft resources (mass, volume and power) available for an EPO camera.

No imaging was obtained during Galileo’s end-of-mission impact into Jupiter. This allows almost an order of magnitude improvement in resolution compared with Galileo’s best, which is in the range 20–25 km (Little et al. The orbit enables observation of the poles at low emission angles, and features close approaches to Jupiter-about 5000 km above the cloud tops at perijove. The orbit plane is nearly perpendicular to the Sun-Jupiter line, so the spacecraft is generally flying along the terminator. The orbital inclination is high, and the closest point in the orbit, perijove, is near the equator. So a visible camera, Junocam, was included primarily for education and public outreach (EPO), funded from the mission’s EPO budget and given a fairly constrained allocation of spacecraft mass resources.ĭespite the challenges, Juno’s polar orbit offers a unique vantage point for imaging Jupiter compared to other missions that have orbited or flown by Jupiter. But it was appreciated that visible imaging is an important component of public engagement for any mission. Such a platform presents challenges for imaging, both from motion blur and pointing geometry. The spacecraft has been highly optimized for the operation of its seven science instruments, leading to a solar-powered, sun-pointing, spinning design. The scientific themes of the Juno mission are to study the interior, atmosphere, and magnetosphere of Jupiter (Bolton et al., this issue).
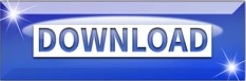